Difference Between Cotransport and Countertransport
Cotransport and Countertransport are two crucial mechanisms that play a significant role in this intricate process. In this article, we will delve into these transport mechanisms, their differences, and their essential functions within the cell.
What is Cotransport?
Cotransport, also known as coupled transport or secondary active transport, is a cellular transport process that involves the simultaneous movement of two or more different solutes across a cell membrane. This process relies on the energy stored in the concentration or electrochemical gradients of certain ions, which are established by primary active transport processes, such as the sodium-potassium pump (Na+/K+-ATPase) or proton pumps.
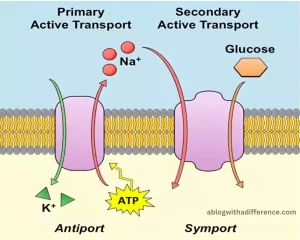
There are two main types of cotransport:
1. Symport: In symport, two or more solutes are transported in the same direction across the cell membrane. One solute moves down its concentration gradient, providing the energy required to drive the movement of the other solute against its concentration gradient. An example of symport is the sodium-glucose cotransporter (SGLT), which transports glucose and sodium ions together into cells.
2. Antiport: In antiport, two or more solutes are transported in opposite directions across the cell membrane. As one solute moves into the cell, the movement of the other solute out of the cell is facilitated. The energy required for this countertransport is provided by the movement of ions along their electrochemical gradients. An example of an antiport is the sodium-calcium exchanger (NCX), which moves calcium ions out of the cell while simultaneously moving sodium ions into the cell.
Cotransport is crucial for various physiological processes, including nutrient absorption in the intestines, reabsorption of filtered substances in the kidneys, and the functioning of nerve and muscle cells. Specific transporter proteins embedded in the cell membrane facilitate the cotransport of solutes, and their activity can be regulated to meet the cell’s needs and maintain cellular homeostasis.
By utilizing the energy from existing gradients, cotransport ensures efficient nutrient uptake and ion balance in cells, contributing to proper cellular function and overall organismal health.
What is Countertransport?
Countertransport, also known as exchange transport or antiport, is a type of cellular transport process that involves the simultaneous movement of two or more different solutes across a cell membrane in opposite directions. Unlike cotransport, where solutes move in the same direction, countertransport involves the exchange of solutes between the inside and outside of the cell or between different cellular compartments.
Important to bear in mind when moving solutes within cells: they will always accompany an outgoing movement of solute. The energy required for this exchange is derived from the existing electrochemical gradients established by primary active transport processes, such as the sodium-potassium pump (Na+/K+-ATPase), or other cellular transport mechanisms.
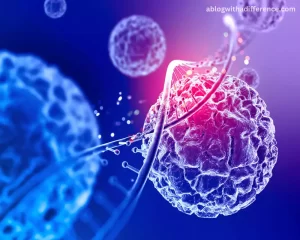
Countertransport can be classified into two main types:
1. Exchange Diffusion: In this type of countertransport, two solutes move across the membrane passively, driven by their respective concentration gradients. One solute moves down its concentration gradient while facilitating the movement of the second solute in the opposite direction.
2. Exchange Pump: In this type of countertransport, the movement of one solute is coupled to the movement of another solute against its concentration gradient. The energy required for this exchange is obtained from the electrochemical gradient established by primary active transport processes.
Countertransport processes play an integral part in many physiological functions, such as homeostasis of ionic concentrations and pH regulation as well as supporting nerve and muscular cell functioning. For example, the sodium-calcium exchanger (NCX) is an essential counter transporter that helps maintain calcium ion concentrations in cells by exchanging calcium ions for sodium ions. This process is crucial for muscle contraction, nerve cell signaling, and other cellular functions.
Dysfunctional counter transporters can lead to various medical conditions. For instance, mutations in certain countertransport proteins can result in ion channelopathies, affecting ion balance and leading to diseases like cystic fibrosis and Bartter syndrome.
Understanding countertransport and its regulation is vital for comprehending cellular physiology and the development of therapeutic interventions for various medical conditions related to ion transport and pH regulation.
Importance of cotransport and countertransport in cellular function
Cotransport and countertransport are both vital transport mechanisms that play essential roles in cellular function. They are responsible for the movement of ions and molecules across cell membranes, allowing cells to maintain proper ion concentrations, nutrient uptake, waste elimination, and overall cellular homeostasis. Below are the key importance of cotransport and countertransport in cellular function:
1. Nutrient Absorption and Reabsorption: Cotransport mechanisms, especially symporters, facilitate the uptake of essential nutrients, such as glucose, amino acids, and ions, from the extracellular environment into the cell. Sodium-glucose transporters (SGLT), present on intestinal epithelial cell membranes, play an integral part in transporting digested glucose back into circulation after it has been digested.
In the kidneys, cotransport processes are responsible for the reabsorption of filtered substances, ensuring that valuable nutrients and ions are not lost in urine.
2. Ion Homeostasis: Cotransport and Countertransport work together to maintain an ideal equilibrium among various ions such as sodium, potassium, calcium (Ca2+), chloride (Cl+), and other components inside and outside cells. Ion concentrations are critical for various cellular processes, including membrane potential, nerve impulse transmission, muscle contraction, and osmotic balance.
3. pH Regulation: Countertransport processes, such as the chloride-bicarbonate exchanger, are essential for regulating intracellular and extracellular pH levels. This is crucial for maintaining the optimal pH environment required for enzymatic activities, cellular signaling, and overall cellular function.
4. Nerve Cell Function: Cotransport and countertransport mechanisms are fundamental for the proper functioning of nerve cells (neurons). They are involved in establishing and maintaining the resting membrane potential and play a crucial role in generating action potentials for nerve impulse transmission.
5. Muscle Contraction: Proper ion balance, facilitated by cotransport and countertransport processes, is crucial for muscle cell function. Calcium ion transport, for example, is vital for initiating muscle contraction and relaxation.
6. Waste Elimination: Countertransport mechanisms, such as the sodium-hydrogen exchanger (NHE), help in removing waste products and excess ions from the cell. This is particularly important for maintaining cellular health and preventing the accumulation of toxic substances.
7. Energy Efficiency: Cotransport and countertransport utilize existing electrochemical gradients, established by primary active transport processes, to transport solutes against their concentration gradients. This energy-coupling ensures more efficient use of cellular energy compared to primary active transport, which directly requires ATP hydrolysis.
8. Adaptation to Environmental Changes: The regulation of cotransport and countertransport processes allows cells to adapt to changing environmental conditions and cellular demands. For instance, in response to changes in nutrient availability or osmotic conditions, transporters can be upregulated or downregulated to maintain cellular balance.
Cotransport and countertransport processes are essential for a wide range of cellular functions, including nutrient uptake, ion homeostasis, pH regulation, nerve cell function, muscle contraction, waste elimination, and efficient energy usage. Transport mechanisms play an essential role in maintaining healthy cells, tissues and organisms alike.
Key Differences Between Cotransport and Countertransport
Cotransport and countertransport are two distinct cellular transport processes, and they differ in several key aspects.
Here are the main differences between cotransport and countertransport:
1. Definition and Direction of Transport:
- Cotransport: In cotransport, two or more different solutes are transported simultaneously in the same direction across the cell membrane. This can occur through symport (same-direction cotransport) or antiport (opposite-direction cotransport).
- Countertransport: In countertransport, two or more different solutes are transported in opposite directions across the cell membrane. One solute moves into the cell while another solute moves out.
2. Types of Transporters Involved:
- Cotransport: Cotransport involves transporters that simultaneously bind and transport multiple solutes. These transporters can be symporters (cotransport in the same direction) or antiporters (cotransport in opposite directions).
- Countertransport: Countertransport also involves transporters that can bind and transport multiple solutes, but the key distinction is that the solutes are transported in opposite directions.
3. Net Movement of Solutes:
- Cotransport: In cotransport, the net movement of the coupled solutes is driven by the movement of one solute down its concentration gradient. The energy from this movement is used to transport the other solute against its concentration gradient.
- Countertransport: Countertransport refers to the process by which one solute moves out of its cell in exchange for another and vice versa. This exchange occurs across the cell membrane.
4. Cellular Energy Requirement:
- Cotransport: Cotransport utilizes the energy stored in the electrochemical gradients of ions, which are established by primary active transport processes like the sodium-potassium pump (Na+/K+-ATPase). No direct ATP hydrolysis is involved in cotransport.
- Countertransport: Similarly, countertransport also utilizes the energy from the electrochemical gradients of ions, established by primary active transport processes or other cellular transport mechanisms.
5. Biological Significance and Physiological Roles:
- Cotransport: Cotransport is crucial for nutrient absorption in the intestines and kidney tubules, reabsorption of filtered substances in the renal tubules, and the functioning of nerve cells and muscle cells. It helps in nutrient uptake and maintaining ion balance.
- Countertransport: Countertransport is vital for ion homeostasis, pH regulation, and the functioning of nerve cells and muscle cells. It contributes to maintaining proper ion concentrations and pH levels in cells.
Both cotransport and countertransport play important roles in maintaining cellular homeostasis and facilitating various physiological processes. While cotransport moves solutes in the same direction to facilitate nutrient uptake, countertransport moves solutes in opposite directions to regulate ion concentrations and pH levels. These transport mechanisms are critical for proper cellular function and overall organismal health.
Relevance of Cotransport and Countertransport in Medicine
Cotransport and countertransport have significant relevance in medicine, as they are involved in essential physiological processes critical to human health. Understanding transport mechanisms can have an immense effect on medical research, diagnostics and therapeutic intervention.
Here are several areas in medicine where cotransport or countertransport plays an essential part:
1. Nutrient Absorption and Transport: Cotransport processes play an integral part in digesting nutrients like amino acids and glucose for absorption in the intestinal tract. Dysregulation of these transporters can lead to malabsorption syndromes and nutrient deficiencies, impacting overall health and growth.
2. Kidney Function and Fluid Balance: Both cotransport and countertransport are involved in reabsorption and secretion processes in the kidney tubules. These transport mechanisms help maintain fluid balance, electrolyte equilibrium and the absorption of essential nutrients, ions and water into cells. Dysfunctional transporters can lead to kidney disorders and imbalances in body fluids.
3. Neurotransmission and Nerve Cell Function: Cotransport and countertransport are critical for neurotransmitter uptake and release in nerve cells. Dysregulation of these transporters may contribute to nerve signaling impairment and lead to neurological diseases like epilepsy or mood disorders.
4. Muscle Function and Excitation-Contraction Coupling: Cotransport and countertransport play a role in maintaining proper ion concentrations for muscle contraction. Dysfunctional transporters can lead to muscle weakness, spasms, or disorders like hypokalemic periodic paralysis.
5. pH Regulation and Acid-Base Balance: Countertransport processes are involved in maintaining intracellular and extracellular pH levels.Acidosis and alkalosis may result from improper regulation, leading to acidity levels being too acidic or alkaloid for their organ systems or cell functions to function optimally. Such disorders have serious ramifications for both organ health and cell performance.
6. Ion Channel Diseases: Dysfunctional cotransport and countertransport proteins can lead to ion channelopathies, such as cystic fibrosis, caused by mutations in chloride channels, and Bartter syndrome, resulting from mutations in ion transporters in the kidney.
7. Drug Targets: Cotransport and countertransport proteins are potential drug targets. Modulating transporters can improve absorption or elimination of certain medications, increasing therapeutic efficacy while decreasing side-effects.
8. Cancer Treatment: Some cancer cells overexpress specific transporters to facilitate nutrient uptake and promote cell proliferation. Targeting these transporters can offer new avenues for cancer therapy.
9. Metabolic Disorders: Dysfunctional cotransport and countertransport proteins can lead to metabolic disorders, such as glucose-galactose malabsorption and Hartnup disease, which affect nutrient absorption and metabolism.
10. Transporter-Based Diagnostics: Understanding the expression and activity of cotransport and countertransport proteins can aid in the diagnosis of various diseases, as alterations in transporter function can serve as biomarkers for specific conditions.
Cotransport and countertransport are critical physiological processes that have a significant impact on human health and disease. Their relevance in medicine spans various fields, from gastrointestinal disorders and kidney diseases to neurological conditions and cancer therapy. Continued research into these transport mechanisms holds the potential for developing new treatments and improving patient outcomes in a wide range of medical conditions.
Regulation of Cotransport and Countertransport
The regulation of cotransport and countertransport processes is essential for maintaining cellular homeostasis and adapting to changing environmental conditions. There are various mechanisms available to modulate transporter activity to suit cell needs.
Here are a few key regulatory mechanisms governing cotransport and contratransport:
1. Cellular Signaling Pathways: Cotransport and countertransport protein activities can be controlled via intracellular signaling pathways. Cascades can be set off by hormones, neurotransmitters or any signaling molecules activating or blocking specific transporters. Insulin may increase glucose transporters’ activity to bring blood glucose levels down more effectively.
2. Post-Translational Modifications: Cotransport and countertransport proteins can be modified after synthesis to affect their activity. Transporter function may be altered through posttranslational modifications such as phosphorylation and glycosylation. These modifications can alter the transporter’s affinity for substrates or its cellular localization.
3. Calcium and pH Levels: Intracellular calcium and pH levels can impact cotransport and countertransport activities. Changes in calcium concentration can directly regulate certain transporters, while pH alterations can affect the protonation state of transporter binding sites, influencing their substrate affinity.
4. Osmolarity and Ionic Strength: Changes in extracellular osmolarity and ionic strength can influence transporter activity. These alterations affect the movement of ions across the cell membrane, thereby modulating the driving force for cotransport and countertransport.
5. Gene Expression: The expression of cotransport and countertransport genes can be regulated at the transcriptional level. Cellular needs and environmental cues can trigger changes in gene expression, leading to an increase or decrease in transporter synthesis.
6. Feedback Inhibition: Some cotransport and countertransport systems are regulated through feedback inhibition. The accumulation of end products or downstream metabolites can inhibit the activity of specific transporters to prevent excessive transport.
7. Co-Transported Substrate Concentrations: The concentrations of co-transported substrates can influence the activity of cotransport and countertransport systems. As substrate concentrations change, transporters may be upregulated or downregulated to maintain the appropriate balance.
8. Cellular Compartmentation: The distribution and activity of cotransport and countertransport proteins in different cellular compartments can be regulated. Transporters may be selectively expressed in specific regions of the cell, allowing for specialized functions in different cellular processes.
9. Adaptation to Environmental Changes: Cotransport and countertransport systems can adapt to changing environmental conditions. For instance, exposure to different nutrient concentrations or ion gradients can lead to adjustments in transporter activity.
The regulation of cotransport and countertransport processes is a complex and dynamic aspect of cellular physiology. The ability to modulate these transport mechanisms allows cells to maintain proper ion balance, nutrient uptake, pH regulation, and overall cellular function, ensuring their survival and proper functioning in various physiological contexts.
Similarities Between Cotransport and Countertransport
While cotransport and countertransport are distinct cellular transport processes with key differences, they also share some similarities.
Here are the similarities between countertransport and cotransport:
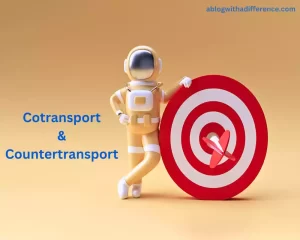
1. Transporter Proteins: Cotransport and antitransport processes rely on transporter proteins located within a cell’s membrane for operation. These transporter proteins are integral membrane proteins that facilitate the movement of solutes across the lipid bilayer.
2. Facilitated Transport: Cotransport and countertransport are both examples of facilitated transport, meaning that they require the assistance of transporter proteins to move solutes across the cell membrane. Facilitated transport allows solutes to move down their concentration gradients or along electrochemical gradients.
3. Active Transport Coupling: While cotransport and countertransport are considered secondary active transport processes, they both rely on the energy derived from the concentration or electrochemical gradients of other ions to drive the movement of the transported solutes. This is in contrast to primary active transport, where energy is directly derived from ATP hydrolysis.
4. Cotransport and Countertransport Play an Important Role: Both transports and counter transports play an essential part in maintaining cell homeostasis. These cells play an indispensable role in maintaining proper cell and organismal health.
5. Importance in Physiological Processes: Both transport mechanisms are vital for various physiological processes within the body. Cotransport plays an essential role in the absorption of nutrients by our intestinal and renal tissues, and also functions of nerve and muscular cells. Countertransport, on the other hand, is crucial for ion homeostasis, pH regulation, and the functioning of nerve and muscle cells.
6. Electrochemical Gradients: Both cotransport and countertransport rely on the presence of electrochemical gradients across the cell membrane. These gradients are established by primary active transport processes, such as the sodium-potassium pump (Na+/K+-ATPase), which create differences in ion concentrations and electrical potentials inside and outside the cell.
7. Regulation: Both cotransport and countertransport processes can be regulated to match the dynamic needs of the cell and maintain cellular equilibrium. The activity of the transporter proteins involved in these processes can be modulated by various cellular signals and factors.
While cotransport and countertransport are different in terms of the direction of solute movement and net transport of solutes, they share common characteristics. Both processes involve specific transporter proteins, utilize facilitated transport, rely on the energy from electrochemical gradients, and play critical roles in maintaining cellular homeostasis and supporting various physiological functions.
Conclusion
Cotransport and countertransport are fundamental cellular transport processes that enable the movement of ions and molecules across cell membranes. While they have distinct mechanisms and directionality of solute movement, they share common characteristics and play critical roles in maintaining cellular homeostasis and supporting various physiological functions.
Cotransport involves the simultaneous movement of two or more solutes in the same direction or opposite directions, driven by the energy stored in existing concentration gradients or electrochemical gradients established by primary active transport processes. This process is crucial for nutrient absorption, reabsorption of filtered substances, and the functioning of nerve and muscle cells.
On the other hand, countertransport involves the exchange of solutes in opposite directions, utilizing the energy from existing electrochemical gradients. Countertransport plays a vital role in ion homeostasis, pH regulation, and the functioning of nerve and muscle cells.