A brief introduction to Baryons and Mesons
Mesons and baryons differ primarily in that baryons consist of three quark particles while mesons consist of quark-antiquark pairs. Mesons and Baryons are two subatomic particles belonging to different families: baryons fall under fermions while mesons belong to bosons; both belong to the hadronic family as a whole and represent intermediate mass particles that makeup Atoms. All particles are composed of quarks. Quarks form the core building blocks that compose matter; therefore they constitute fundamental particles.
Importance of understanding the difference between baryons and mesons
Understanding the difference between Mesons and Baryons is of crucial importance when it comes to particle Physics.
Here are several reasons why it is crucial to comprehend their distinctions:
1. Particle Classification: Both baryons and mesons fall under the larger category of hadrons – particles with powerful nuclear forces – so categorizing and classifying different particles helps provide insight into what makes up matter itself. Baryons and mesons help distinguish this division further and shed light on what composes it.
2. Structure of Matter: Baryons such as neutrons and protons form the essential building blocks of nuclei in atoms, providing structural stability and properties of these atoms. Acknowledging their characteristics and behaviors is vital in understanding how matter structures itself both on a subatomic and atomic scale.
3. Strong Nuclear Force: Mesons play an indispensable role in mediating nuclear force, commonly referred to as strong interaction or strong force. The strong interaction or strong force is responsible for binding neutrons and protons together in atomic nuclei; studying mesons provides new insight into its mechanism, offering a new understanding of nuclear nuclei physics as well as behavior.
4. Quantum Dynamics: Baryons and mesons both consist of quarks, the fundamental particles. By studying differences between mesons and baryons, scientists gain valuable insight into quark dynamics – for instance, confinement/interaction patterns for quarks within hadrons as well as any principles regulating their behavior within hadrons – providing further knowledge of particles/forces which compose our universe.
5. Particle Interactions: Baryons and mesons play an essential role in many-particle interactions, with baryons particularly playing a pivotal role. By studying their unique properties and behaviors, scientists can investigate mechanisms such as decay and scattering which occur between particles during interactions; such knowledge provides invaluable insight into interpreting data from experiments as well as improving our knowledge of fundamental particles and forces that make up our universe.
Understanding the difference between mesons and baryons is fundamental for classifying particles, understanding matter structures and nuclear forces, as well as studying quark dynamics and particle interactions. Scientists continue pushing the limits of particle physics knowledge while deepening our knowledge about what makes up our universe.
What Are Baryons?
Baryons are subatomic particles that contain three distinct sizes of quark particles. As fermions with half-integer spin, baryons fall under their umbrella category of fermions. Since baryons contain quarks they may participate in strong interactions such as strong nucleus forces; two such examples would be protons and neutrons.
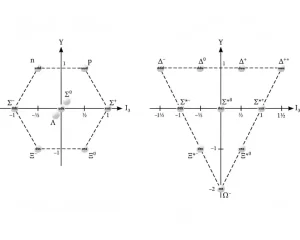
As these particles contain three quarks, we often refer to them as baryon “triquarks”. Baryons tend to be massive particles compared to other subatomic particles; other examples are lambda, sigma, and xi particles as well as omega particles.
Alongside their spin and charge numbers, particles have two quantum numbers we could assign them: baryon numbers (1 = baryons), and strangeness. Strangeness is measured using “strange quarks”. Baryons make up most of the visible matter.
Protons and neutrons are considered baryons; these particles make up the primary constituents of atoms – the most compact elements on Earth. Electrons do not belong to this same family of particles known as “baryons”, however. Instead, they belong to another known as “leptons”. Leptons differ from baryons by not being able to interact through force alone.
What Are Mesons?
Mesons are subatomic particles consisting of both quarks and antiquarks. Bosons classify meson particles, and all mesons tend to degrade over time into neutrinos or electrons if charged mesons exist.
Uncharged mesons decay to form photons through decay. Mesons possess integer spin (baryons have half-integer spin). Mesons come in various sizes with the pion being the smallest one. Mesons participate in both strong and weak interactions while charged versions may even take part in electromagnetic interactions as well.
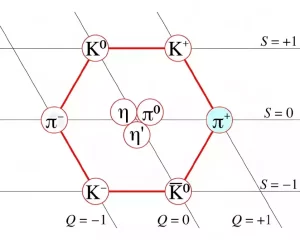
Mesons can be classified according to their quark content, total parity, and angular momentum. Although all mesons can be unstable, those with lower masses tend to be safer than larger ones.
Difference Between Baryons and Mesons
Mesons and baryons differ primarily in their composition; we will investigate further their structures below:
Baryons:
Baryons are composite particles composed of three quarks bonded together through nuclear force and are the fundamental particles that exist throughout nature.
Each quark has different qualities ranging from up, down, and mysterious charm – often described as being “up”, “down” and mysterious charm; in various combinations, these flavors of three quarks result in different kinds of baryons (protons are composed of two up quarks with an additional down quark (uud), while neutrons contain two down quarks plus one up.
Once combined these three quarks create the unique characteristics associated with that specific baryon – mass, charge, spin as well as spin properties (and spin!).
Mesons:
In contrast with baryons, mesons consist of two quarks and an antiquark pair. An antiquark is a counterpart to every quark; its mass is identical but with different charge and quantum characteristics; mesons can contain various combinations of quarks and antiquarks ranging from those like anti-down and down or bizarre and odd (s S), producing various properties when bound together as one solid form.
Mesons and baryons are composite particles, meaning that they are created through the combination of two essential particle types (quarks or antiquarks) to form new entities with their interactions, characteristics (mass, charge, spin, and decay patterns) shaped by these combinations affecting how these particles behave as whole entities.
Scientists can gain invaluable insight into quark dynamics, strong force, and how matter is structured at the subatomic scale by examining mesons and baryons compositions.
Mass and Spin:
Mass and spin are important characteristics that differentiate baryons and mesons. Let’s explore how mass and spin vary between these two classes of particles:
Baryons:
In contrast with mesons, baryons tend to possess greater masses due to containing three quarks that contribute significantly to their mass. Different baryons may vary slightly in terms of mass depending on which quark flavors they possess; protons and neutrons – two popular baryons – possess slightly different masses due to variations between down and up quark masses present within them.
Concerning their spin, baryons can possess half-integer values like 1/2/3/2/5/2 and so forth. A particle’s inherent angular momentum plays a central role in its behavior and interactions; hence the half-integer spin values found in baryons are due to three quarks having an angular momentum of 1/2 and their combination to produce overall spin values of half an integer value.
Mesons:
In general, mesons tend to have lower masses than baryons due to being an antiquark-quark pair whose mass is determined by both elements’ masses; for instance, pions have the lowest masses as their composition varies based on whether it consists of anti-up or up quarks (p+), up and down quarks (p0), bizarre and odd quarks (K mesons), etc.
Mesons exhibit integer spin values that range between zero, one, two, or three due to interactions between quarks and antiquarks; each having an average spin of 1/2 which creates an infinity multiple spin value for total meson spin values.
Understanding the spin and mass characteristics of mesons and baryons will provide valuable insight into their structure, internal relationships with other particles, and behavior across physical phenomena. Furthermore, such properties play a major role in particle physics research that studies different particle spins and masses to discover the fundamental principles and mechanisms that control the subatomic world.
Electric Charge:
The electric charge is another important aspect that distinguishes baryons and mesons. Let’s examine how electric charge varies between these two classes of particles:
Baryons:
Baryons contain negative, positive, and neutral electrical charges depending on which quarks reside within them. Protons, members of the baryon family, have positive charges composed of two up quarks (with +2/3 charges each) and one down quark with charges equal to 1/3.
Protons contain five quark charges which create a net cost of one, while neutrons, as second baryons, have no net charge due to being composed of three low-charge quarks (1/3 charge each) plus one higher-charged quark (+2/3).
Mesons:
In contrast with baryons, mesons typically possess no electric charges at all. Mesons have zero charges since their composition consists of two quarks and an antiquark pair each possessing different electric charges; their electric charges combine and cancel out to create zero net electric charge in mesons.
A pion or meson particle consists of both up quarks (charge of +2/3) and anti-down quarks (charge -2/3) which combine into an electrical charge equaling zero, producing an electromagnetic force called the electric field.
Understanding the electric charge of baryons and mesons is vital to understanding their interactions with electromagnetic fields as well as their behavior in various particle interactions.
Scientists use electric charge knowledge to study particle reactions and understand their role in subatomic particle interactions, while it also assists them with distinguishing between particles based on their characteristic charge. Knowing about electric charge also aids them in distinguishing different species based on their particular charge characteristics.
Lifetime and Decay:
Mesons and baryons possess distinct properties when it comes to their decay and life span, making their study essential to particle physics. Let’s compare and contrast the properties of decay and lifetime between mesons and baryons:
Baryons:
In general, baryons are considered stable particles. Their long lives and lack of spontaneous decay stem from their nature: nuclear force binds quarks together tightly enough that there’s little chance they’d disassemble spontaneously – acting like an anti disruption force that keeps quarks together instead of breaking. Therefore, baryons tend to exist for longer before decay occurs – providing ample time for exploration!
At the same time, however, it’s essential to recognize that some baryons can decay over time through weak interactions rather than strong forces; neutrons that do not reside inside an atomic nucleus may experience decay caused by weak interaction and have an estimated 14-minute lifespan before dying off completely.
Mesons:
Mesons differ from baryons by having very short lives and decaying spontaneously. Their short duration is due to two quarks and an antiquark pair bound by an electromagnetic force, rather than three like baryons are. Thus mesons are more vulnerable to decay.
Mesons decay by various means, including weak and strong decays. Weak decays involve the conversion of one type of quarks or antiquarks into another type mediated by strong nuclear forces; strong decays involve an unstable transition.
Strong decays occur when meson quark and antiquark pairs are destroyed, creating new particles such as baryons or mesons. Their specific decay channels and lifetimes depend on various properties including mass, composition, and quantum numbers.
Understanding the decay and lifetime properties of mesons and baryons can yield valuable insight into the subatomic dynamics of particle physics, their interactions, forces that govern them, fundamental processes taking place at subatomic levels, as well as scientific processes happening on smaller scales.
Scientists can study mechanisms through which particles change or decay to further their knowledge of particle physics as well as matter itself on smaller scales.
Comparison Chart of Baryons and Mesons
Here’s a chart of comparison that highlights the major differences between mesons and baryons:
Characteristics | Baryons | Mesons |
---|---|---|
Composition | Three quarks | Quark-antiquark pair |
Examples | Protons, neutrons, lambda baryon, sigma baryon | Pions, kaons and rho meson J/psi meson |
Mass | The mesons have generally higher mass | In general, baryons have lower mass than baryons. |
Spin | Half-integer spin values | Integer spin values |
Electric Charge | Positive, negative, or neutral charges | Neutral charges |
Lifetime | Life expectancy is relatively long | A relatively short life span |
Decay | Most stable, they do not undergo spontaneous decay | Can undergo spontaneous decay processes strong |
Strong Interaction | The strong nuclear force holding | Holding together by the powerful nuclear force |
Quark Configuration | The Three Quarks (combinations of down, up or strange quarks) | Quark-antiquark pair (e.g., up-antidown, up-antistrange) |
Interactions with Gluons | Participate in gluon exchanges and interactions with strong forces participate | Participate in gluon exchanges and strong force interactions |
The Role of Atomic Nuclei | The nucleus is formed and contributes to its stability | Indirectly involved in atomic nuclei |
Strong Interaction
The powerful interaction, also referred to as the nuclear force strong, is one of the four fundamental forces defining our natural world. This force is responsible for keeping nuclei of atomic energy together as well as playing an essential role in baryons’ and mesons’ behavior and characteristics.
Let’s explore this idea further!
1. Binding Force: Powerful interactions refer to forces that bind quarks in baryons (such as neutrons and protons) together and keep them in their places, binding quarks with antiquarks to form mesons. This force, commonly referred to as the strong one, acts at extremely short distances; far more so than electromagnetic force.
2. Gluons: Gluons provide powerful forces by acting as force carriers between quarks, similar to how photons carry electromagnetic radiation’s force. These exchange particles move the strong forces between quarks, acting like photons in electromagnetic radiation’s transmission process. Gluons interact with quarks to transfer strong forces while helping keep quarks cohesive in Hadrons (baryons and mesons).
3. Quantum Charge (Color Charge): Quarks are elementary particles found within mesons and baryons that possess an intrinsic property known as a color charge, similar to electromagnetism’s electric charge but with three possible values: green, red, and blue – as well as their antired counterparts (antigreen being red in this instance). Quarks interact through electron exchange which transports this charge of color between one another.
4. Confinement: One of the hallmarks of power is quark confinement, an effect in which quarks always exist within hadrons (baryons and mesons), never free floating as individual particles. Instead, due to strong forces preventing quarks from moving freely apart from each other as free particles. Whenever quarks are isolated individually from their hadronic hosts they accumulate energy until it becomes optimal to generate new bound states instead of isolating individual quarks separately.
5. Asymptotic Freedom: While quarks tend to remain tightly bound at lower energies, strong interactions exhibit an intriguing property known as Asymptotic Freedom at higher energies. At extreme energies, the strength of forces diminishes and quarks become freer to interact. This property was discovered via quantum chromodynamic theory (QCD), one key feature characteristic of strong forces.
Understanding interaction strength is vital to understanding hadrons’ behavior, nuclei’s structure and composition and quark and gluon composition and behavior, and their evolution over time. Interaction strength also plays a key role in baryons’ creation, stabilization, and decay processes and their subatomic realm regulation; its understanding forms part of understanding fundamental forces governing subatomic realm dynamics.
Role in Particle Physics
The strong interaction is an indispensable aspect of particle physics. It contributes to our knowledge of elementary particles as well as the forces affecting them, providing invaluable insights into their behavior.
Here are the key roles it serves within particle physics:
1. Hadron Structure Strong Interaction: the strong interaction is what gives hadrons their stability and structure, such as baryons (such as neutrons and protons) as well as mesons. It connects quarks as well as antiquarks inside these particles to allow composite structures to form; studying this interaction helps us understand inner dynamics as well as the composition of hadrons.
2. Quark Confinement: Quark confinement is one of the more fascinating aspects of powerful interaction, where quarks remain bound within hadrons rather than appearing free as particles that move around on their own. Strong forces serve as primary agents behind quark confinement. Understanding this phenomenon is crucial to comprehend why we don’t encounter isolated quarks, as well as comprehending characteristics associated with hadrons.
3. Quark-Gluon Plasma: Under extreme temperatures or energies similar to those encountered in particle colliders or at the beginning of the universe, strong interactions become extended; quarks and gluons no longer form part of hadrons but instead form their quantum of matter known as quark-gluon phenomenon. Understanding its characteristics provides insight into particle behavior in extreme conditions.
4. Strength Nuclear Force: Powerful interactions are at the root of the strong nuclear force that binds protons and neutrons in nuclear nuclei together, creating strong nuclear interactions. Understanding this force enables us to comprehend the stability and characteristics of nuclei made up of atoms; an understanding which is indispensable in terms of nuclear physics, nuclear energy production, and understanding processes in stars like star fusion.
5. Lattice QCD: The strong interaction is difficult to analyze analytically due to its complexity, so quantum chromodynamics in the lattice (QCD) serves as an indispensable instrument in understanding its strength. Lattice QCD uses supercomputers to model quarks and gluons on discrete grids of space-time discrete grids, providing important insight into the physical properties of hadrons and powerful forces.
6. Symmetry Breaking: Interactions with the Higgs field play an integral part in electroweak symmetry breaking, giving masses to elementary particles like quarks and leptons while providing support for the Standard Model of particle physics.
Strong forces are an integral component of particle physics helping us gain insight into the nature and behavior of hadrons as well as understanding the forces that shape the Universe. By studying strong interactions scientists can better understand fundamental particles’ behavior and unravel all the mysteries hidden deep within subatomic space.
Experimental Evidence
Strong interactions, which play a vital role in nature, have been extensively researched and verified through various experiments and evidence.
Here is some notable experimental proof supporting their existence and nature:
1. Scattering Experiments: Studies that involve high-energy particles colliding with targets have provided compelling evidence of the strength of interaction. These experiments show how particles behave under strong forces as well as exchanging gluons; their scattered paths at various angles and energies indicate the existence of powerful forces which direct their interactions.
2. Engaging Inelastic Scattering: Studies by Stanford Linear Accelerator Center (SLAC) during the 1970s and 1960s provided direct evidence of quarks within nucleons (protons and neutrons). Experiments included firing high-energy electrons at nucleons before studying their scattered electrons or any particle emissions by them, with results showing patterns of scattering that proved the existence of quark constituents within hadrons.
3. Jet Formation: Particle colliders such as the Large Hadron Collider (LHC) have revealed evidence of particle jet formation during high-energy collisions. Jets are collimated spouts of particles created from the fragmentation of gluons and quarks during a collision, providing further proof of strong forces at work in hadronization, Parton showering processes controlled by strong interactions like this one.
4. Meson and Baryon Spectroscopy: Experimental investigations of energy spectrum resonances have provided new insight into their properties, interactions, and nature of strong interactions among mesons and baryons. By looking at the mass, spin, and decay styles of various mesons and baryons researchers have verified both its presence and power to dictate physical characteristics.
5. Lattice QCD Calculations: Lattice quantum chromodynamics (QCD) is a numerical method that simulates the behavior of gluons and quarks on a discrete grid structure, providing significant insight into strong interactions. By employing lattice QCD calculation experts can precisely compare experimental observations such as hadron masses and interactions, correlating predictions with measurements as proof of both the existence and accuracy of strong interactions.
6. Nuclear Physics: Examining nuclear nuclei and their interactions is one avenue by which evidence for strong interaction can be discovered through experiments. Nuclear properties like binding energies and decay rates as well as reactions are indicators that strong forces exist, with consistent experimental results with theoretical models that incorporate this force further bolstering its credibility.
These scientific pieces of evidence and theoretical advances have furthered our knowledge of interaction’s strength as a fundamental force of nature. They support the existence of gluons, quarks, and mechanisms governed by forces governed by nature which support quantum chromodynamic theories (QCD) as well as our appreciation of strong interactions in the subatomic realm.
Conclusion
Understanding the differences between mesons and baryons is vital to comprehending particle physics. Baryons such as neutrons and protons contain three quarks while mesons consist of quark-antiquark pairs; their properties vary with regards to spin, mass, electric charge as well as lifespan and decay properties. Baryons tend to possess larger masses and half-integer spin values and may exhibit positive neutral or negative electric charges depending on their composition.